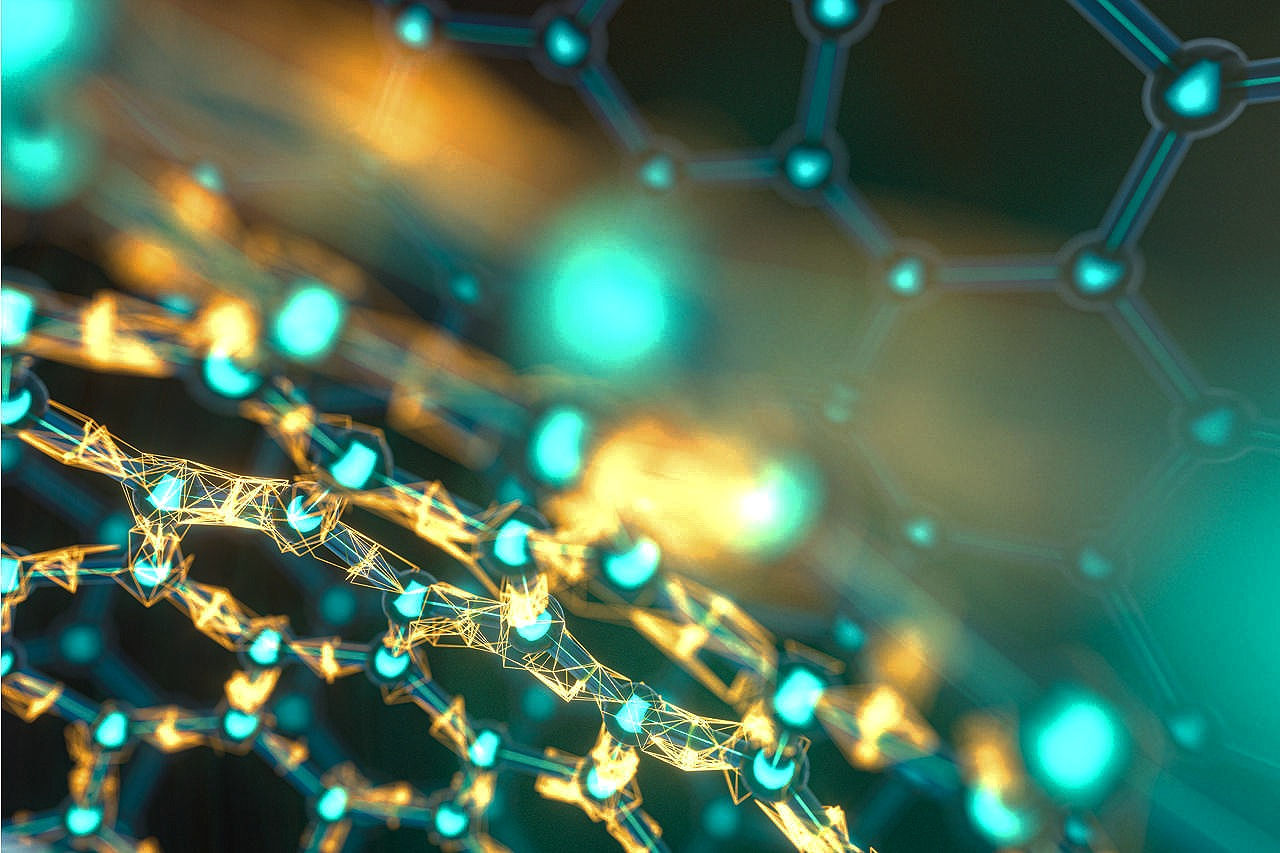
DESIGN NUCLEIC ACID & DRUG DELIVERY SYSTEM
With the fusion of Technological Engineering and Biology, Synthetic Biology is boldly advancing towards dealing with complex ‘never dealt before’ problems. The range extends from building novel bio-components to rewiring the body’s original physiology. The introduction of genetic engineering techniques like Recombinant DNA Technology has seen the light for almost half a century.
Since then, fast-paced advancements in science have been observed. With technologies aiming for the genes, pathways, and proteins of living organisms, Synthetic Biology has successfully taken a giant leap towards broadly dealing with the Cell Bio-components like RNA, DNA and using that knowledge to construct Medical Devices, Transcriptional Factors, and more.
​
To understand the design of Nucleic acid with the help of synthetic Biology, one should understand what synthetic nucleic acids are. Synthetic nucleic acids, according to the NIH guidelines, are molecules that are chemically or by other means synthesized or amplified. It also includes those that are chemically or otherwise modified but can base pair with natural nucleic acid molecules.
​
Designing nucleic acid is very important as it not only paves a path for other niche areas like DNA nanobiotechnology but also helps in studying secondary structures and the target sites more clearly. The principle of synthetic Biology lies in harnessing the properties of the central dogma and making it useful for humans and the biotechnological sector.
During the designing of nucleic acid, the main focus is to deliver de novo DNA/ RNA synthesis that can improve the traditional genetic recombinant technology and overcome limitations in the native cell machinery.
​
System biology doesn’t need to engineer cellular systems to use pre-existing DNA as a template when designing and engineering DNA. It uses the building blocks to create de novo DNA that has more novel functions than ever witnessed in the natural environment. Even though the aim is clear, it isn’t an easy process.
For the DNA to work, it should have operons and other components that don’t get negatively influenced by the host cell machinery. Designing the nucleic acid doesn’t only stop at DNA and RNA synthesis. It also includes the engineering of transcription and translation processes.
The majority of nucleic acid analogs that were developed over the past few decades were made for medicine, drug discovery, and development. Such molecules were designed to inhibit cellular machinery associated with the progression of a disease.
One of the techniques to bring desired changes is to introduce analogs that create bonds and other chemical linkages similar to the original ones. The different types of analogs copy the shape and polarity of the natural bases and maintain the Watson–Crick-like network of hydrogen bonding between nucleotide bases. The truly interchangeable part at the molecular level deals only with nucleic acids whereas, using amino acids and protein secondary structures as interchangeable parts have not been possible yet.
​
It is believed that the synthetic efforts will install the predictability and reliability to engineered biological systems just like chemical engineering bringing to chemical systems. That being said, the ability to sequence DNA is currently better than synthesizing DNA de novo.
​
Let’s look at the step-wise production process of designing a nucleic acid. (photo)
​
I) The current scenario of engineering biological systems requires many steps of trial and error to investigate and evaluate novel enzymes, expression systems, and pathways for the desired function. Typically, this process starts by designing a desired synthetic biological circuit or pathway using computer-aided design (CAD).
ii) Then the resulting synthetic construct DNA is divided into smaller overlapping pieces of around 0.2-1.5 Kbp segments that are easier to synthesize and are called synthons.
iii) These DNA components (synthons) are then chemically synthesized from a set of overlapping single-stranded oligonucleotides by commercial vendors.
iv) The resulting overlapping synthons are then introduced into larger pieces of DNA assemblies or devices and then cloned into an expression vector and the sequence of the resulting construct is then verified and studied.
v) The sequence-verified DNA constructs are then transformed into a cell and assayed for function.
​
Depending on the test results, changes can be made to the design, and further iterations of the test cycle can be repeated. The process has become the backbone of synthetic biology, and so it drives the automated processes and methods that can shorten the development cycle, increase throughput and prove economic efficiency.
​
To date, there have been a handful of examples and demonstrations like the complete synthesis of an entire yeast chromosome, an entire bacterial genome, and the subsequent synthesis of a minimal bacterial genome. These examples tell us about the use of synthetic DNA and the potential of existing gene synthesis methods to carry large-scale synthetic biology projects.
Chemical synthesis of DNA oligonucleotides and their assembly into synthons, genes, circuits, and full genomes is possible with the help of advancing gene synthesis technology. Still, the implementation into larger DNA constructs is yearning for better efficiency. With all these successful gene synthesis technologies, the focus is to implement synthetic constructs into a generic host that is completely accepted by the host.
This will allow it to rewrite and rewire the cell’s program, reduce the cost of the entire process by using oligonucleotide columns or microarray-based oligonucleotide synthesis, increase the throughput and improve the quality of the synthetic DNA.
​
​